The aurora borealis is a beautiful and unusual phenomenon. And as is often the case – its explanation is both simple and complicated….
If you want to understand the unusual and fascinating phenomenon of the aurora borealis – this article is exactly for you. However, if you just want to see the northern lights upon your visit to Iceland, everything you need is described in detail in a separate article: Hunting Aurora in Iceland – Complete Guide.
What is Aurora Borealis
V
iking people believed that the aurora borealis is the light reflected off the armor of the Valkyries preparing – along with Odin – for Ragnarok. According to some legends, it was also a bridge leading the fallen warriors to Valhalla.
In Icelandic folk tales, the aurora was said to facilitate childbirth, but pregnant women should not look at it lest the baby be born cross-eyed…
In the short, modern, scientific terms, aurora is the glowing of the atmosphere particles (mostly nitrogen, but also oxygen) due to collisions with extremely fast electrons “shot” from the Sun.
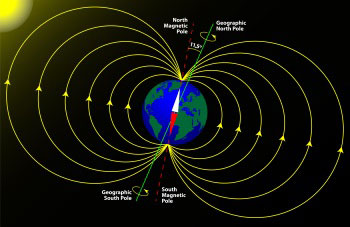
Earth’s magnetic field
These electrons, in waves called the “solar wind” arrive to Earth across its length and width, but the planet’s magnetic field “pushes” them toward the poles or more specifically – to the Arctic Circles. Only there, near the Arctic Circles, can solar particles penetrate our atmosphere, collide with its atoms and cause them to glow.
Electrons forming the solar wind concentrate in waves created during so-called coronal mass ejections (CMEs). That is when a large amount of matter is being pushed out from the Sun’s outer layers into space. The source of solar wind can also be the so-called coronal holes (or more commonly: spots on the Sun), but they are of lesser importance.
The mechanism of creating the light of aurora is almost identical to how fluorescent lights, neon lights or popular xenon car headlights glow. Gas molecules receive a dose of energy (like: are hit with hi-speed electrons) and, wanting to return to their original state, they need to get rid of this extra energy, which they do by emitting photons – the light particles.
The main difference is that in our everyday lamps we make neon or xenon glow, while in the aurora, it is the athmosphere gases – so mostly oxygen and nitrogen – that glow. This means that the color and intensity of the light is different, but the fundamental mechanism of creating light is the same.
In
English we speak of Northern Lights and Southern Lights, and in Latin it is Aurora Borealis and Aurora Australis, respectively. The Icelandic the word for aurora is norðurljós, which translates quite directly to Northern Light.
The mechanism of creating light on both “ends” of the Earth is the same, it’s just that in the southern hemisphere it is much more difficult to see as it passes a lot more over the oceans and far away from populated land.
The term Aurora (that’s the name of the Roman goddess of dawn) was first used for describing the phenomena by Galileo Galilei.
If auroras are created by electrons hitting the Earth’s atmosphere, how come they don’t arrive the same way all the time and why can’t auroras be (usually) observed in Rome, Miami or Kuala Lumpur?
The aurora borealis is not always equally strong and you can’t observe it with the same, equal intensity everywhere on Earth due to two main factors. The first one is the variability of the solar wind, so changes in how strong or weak it is. And the second one is the Earth’s magnetic field, which doesn’t cover the planet in the same way everywhere. Let’s deal with the former factor first.
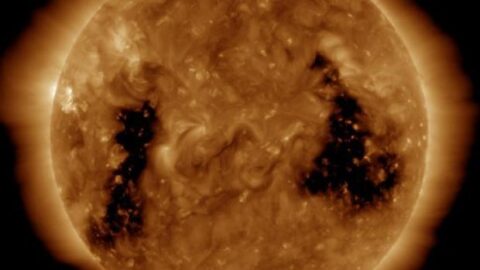
Sun’s turbulent surface [source: www.swpc.noaa.gov]
Our star – the Sun – is a huge ball of helium, in which a thermonuclear fusion reaction is going on all the time. This brings light and heat to Earth, but thanks to the same reaction, “loose” electrons (and other particles) are also sent toward us.
The sun basically sends a fairly uniform amount of these particles in all directions all the time. But that’s “statistically uniform” – it’s quite stable if you average it over a longer period of time, but not so if you compare short time periods. Sun’s natural thermonuclear fusion reaction is an inherently irregular, somewhat chaotic process. Spontaneous crests and troughs (so high and low points) occur in it, which, in addition, do not occur over the entire surface (or volume) of the Sun simultaneously, but rather ‘happen’ occasionally in different places inside and on the face of our star.
One of the effects of this irregularity are eruptions on the surface of the Sun, topped by ejections of huge amounts of solar matter into space. If the location and direction of such an eruption cross paths with Earth’s trajectory, we observe this as a significantly increased ‘density’ and speed of the solar wind.
We
observe
some level of solar wind reaching Earth all the time. It doesn’t stop. And it can easily damage the information stored in our electronic systems, but potentially also damage the systems themselves. All the satellites (so satellite TV, GPS-type systems etc) are at the forefront of this danger, as they are largely not protected by Earth’s magnetic field. But a truly strong wave of solar wind can potentially reach the face of the Earth too, and damage any electronics-based system we use. What’s more: outbursts like that have happened before, but our civilisation lived through them mostly unharmed, because we were not as reliant on electronics, as we are now. A magnetic storm similar to the
Carrington Event from September 1859, could be catastrophic, were it to repeat today.
The effect of the earth’s magnetic field on the aurora
Solar wind particles try to traverse space in a straight line, regardless of which way they are flying or if they encounter a planet or a moon in their path.
But the electrons in that wind are electrically charged, and the Earth, in turn, is surrounded by its own magnetic field. And electrically charged particles in a magnetic field stop moving in a straight line. They start moving along the lines of this magnetic field.
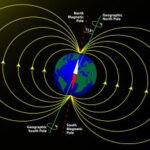
Earth’s magnetic field
Deep inside the Earth there is an iron nucleus. In a huge simplification: a ball of liquid iron. And this iron nucleus creates a magnetic field that looks a bit like if someone stuck a huge magnet inside our planet, mostly aligned with its rotation axis. The lines of Earth’s magnetic field extend above the planet’s surface near the poles (but not at the poles themselves, but rather near the Arctic Circle) and “merge” with lines from the other pole high above the equator.
Earth magnetic field creates a kind of magnetic bubble, cushion or dam over the equator and mid-latitudes. This ‘cushion’ doesn’t reflect the electrons back to the Sun, but rather pushes them aside and towards the arctic circles. The lines of Earth’s magnetic field become something like trail paths for the electrons. The electrons have to follow them. And so near the arctic circles, where these magnetic fields “exit” to the Earth’s surface (or from the electrons’ perspective: go into the Earth), charged particles can – or even must – enter our atmosphere, collide with its gases and make them glow.
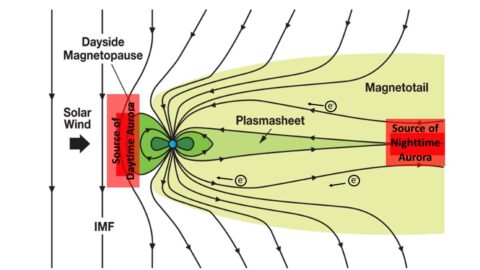
Sources of electrons in the aurora [source: NOAA]
Strictly speaking, as it often happens, this is a bit more complicated. Electrons coming directly from the Sun create magnetic storms in the Earth’s atmosphere, but they do so on the side of the Earth to which they arrive, so basically: the daytime side. We don’t see these storms as auroras, because they happen during the day, when the sky (or rather the Sun) is far too bright for us to see the faint aurora light.
The electrons that form magnetic storms at night, and thus the nighttime aurora instead, come from the tail of the Earth’s magnetosphere. The solar wind accelerates them and forces them into the atmosphere, but strictly speaking these are not electrons arriving “straight from the Sun”. [source: NOAA]
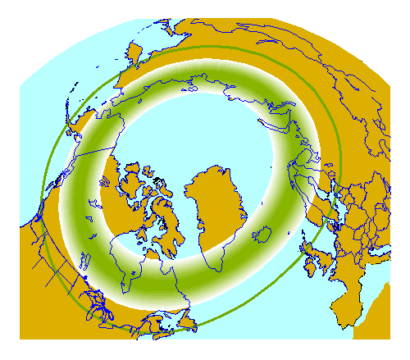
Typical northern hemisphere aurora area. [source: UAF]
And so electrons are being “pushed” into the atmosphere near the Arctic Circle, where they collide with particles in the Earth’s atmosphere and cause them to glow. And this is why the area where the aurora is visible is an oval – a hollow ring. For the same reason, auroras can usually be seen near the Arctic Circle, but not at the pole itself. Although, for the sake of accuracy, let’s add: the center of the aurora oval lies over the Earth’s magnetic pole, which is not exactly the same as Earth’s geographic pole.
In the northern hemisphere, the aurora oval runs through the cold, far north, but nevertheless it is still civilised areas, which we can relatively easily reach. These are Alaska, Canada, northern Sweden, Finland, Norway and Russia, the south of Greenland and virtually all of Iceland. In the southern hemisphere, the aurora borealis appears over the Arctic Ocean and Antarctica, so it is very difficult for people to see it there.
What is the Kp index
The Kp index is an indicator commonly used to determine the severity of global magnetic disturbances in near-Earth space. In other words: to measure the strength of magnetic storms in the atmosphere.
The Kp index was introduced by Julius Bartels in 1938. It was also this German scientist, born in Magdeburg in 1899, who proposed the name and designation of the index. The name Kp comes from “planetarische Kennziffer,” which simply means “planetary index.”
The Kp value is determined as a weighted average of K indices determined at 13 (formerly 11) ground-based magnetic field observatories. Each of these observatories measures its “own” range of magnetic field changes, which are caused by phenomena other than diurnal variability and long-term components of storm time variability. This value is then related to a typical average value for the location. In this way, the K index for a single observatory is determined, and then by averaging the values observed at all observatories, the Kp, or planetary K, is calculated.
Kp
values are determined in 3-hour intervals and range from 0 (very quiet) to 9 (very disturbed). In most aurora forecasts Kp value is given in whole numbers (1, 2, 3, etc.), but at the bottom of it the index values are determined in 28 degrees: 0, 0+, 1-, 1, 1+ …. 9-, 9 and NOAA in its 3-day forecast gives the Kp value to 2 decimal digits – e.g. 4.97.
In Iceland, values below 4 give a chance of seeing a fairly small and weak aurora. Kp between 4 and 6 is already a typical good “solar storm” and a chance for an aurora of full size and beauty. Kp values above 6 allow hunting for the largest and spectacularly beautiful polar lights. Larger Kp also translates into a larger diameter aurora oval – you can then see it further from the pole.
In
practice, chances are you’ll see the aurora in Iceland even when the Kp index is zero. But the higher the Kp value is, the better your chances of seeing aurora large and strong. It can be said that with a value of Kp below 4 you have to be quite lucky to see the aurora, whereas with Kp above 6, you must be terribly unlucky NOT to see it….
How are aurora colors created
The color of the aurora depends primarily on which element is glowing (oxygen or nitrogen) and at what altitude above the Earth’s surface.
N
orthern lights are most often mild green in color (wavelength 557.7 nm). But it can also be blue, purple or red. In contrast, the white aurora borealis is an illusion – a result of imperfections in human vision.
Aurora light is most often formed by oxygen, shining at an altitude of 120 to 300 km. It then gives the popular green light. If you see red aurora (which is rare), it means it is created by oxygen glowing at an altitude of more than 300 km.
Blue, or violet light, on the other hand, is emitted by nitrogen, at altitudes between 120 and 200 km.
Nitrogen emits its light faster than oxygen. Simply put, less time passes from the moment a molecule has accepted energy from a collision with an electron until it gives back that energy by emitting photons. That’s why when we see a combined, violet and green aurora (that is, both nitrogen and oxygen are glowing), we usually get the impression that the violet is leading, preceding the green, which in turn seems to be following it. It’s just that the nitrogen starts glowing there a little earlier than the oxygen.
Morning and evening auroras often have an additional, rather large violet component in their upper part – a kind of a violet plume. It is formed where nitrogen atoms are further stimulated by sunlight. This effect, of course, can only be seen at dusk and dawn – when a little sunlight has already reached (or is still reaching) the upper layers of the atmosphere, but when it is still (or already) dark enough to see the aurora at all.
There is no white aurora, though. If we see the aurora light being white, it means that the strength of its light is simply too weak for our eyes to be able to recognize its color. In very low light, human eyes cannot distinguish between colors and only see in black and white. Modern cameras cannot be “fooled” in this way and will always show us the true color of the aurora in the photo. So in a sense you can verify the color of such a very delicate aurora, by taking its portrait 🙂
How are the aurora forecasts made
As gentle solar winds reach the Earth almost constantly, small, weak aurora borealis can be seen very often in Iceland. In contrast, larger magnetic storms are quite rare and difficult to predict.
Scientists make four types of aurora forecasts. These are published by the U.S. Space Weather Prediction Center (Space Weather Prediction Center, under the National Oceanic and Atmospheric Administration) and the University of Alaska at Fairbanks, among others.
Long-term forecasts
The multi-year forecast is based on inference from the solar activity cycle – how often CMEs and sunspots occur on the Sun. This cycle lasts about 11 years, so there are five to six years between its maximum and minimum. In the period of about four to five years around the maximum, magnetic storms occur more frequently and are more intense. The peak of the current cycle is expected to fall in late 2024 and early 2025, so at the time of writing this article we are perfectly positioned for great aurora spectacles. Unfortunately, it is not possible to deduce from this multi-year forecast a specific date and location of a large, strong aurora.
27-day forecast
The largest auroras are caused by explosions that eject billions of tons of plasma from the Sun into space – these are Coronal Mass Ejections or CMEs for short. These eruptions, once started, are fairly stable on the face of the Sun and often last for up to many months. So it’s relatively easy for scientists to keep track of them.
The combined rotational motion of the Earth around the Sun and the rotational motion of the Sun around its own axis cause a given spot on the Sun’s surface to “look” (or “shoot”) at the Earth about every 27 days. Therefore, if we record a particularly strong solar wind once, we can expect to record it again after about 27 days. In this way (simplified, of course), a 27-day forecast is created.
Unfortunately, the accuracy of this forecast is rather low. By its very nature, this forecast does not take into account new CMEs, historical peaks can shift in the forecast even by a few days, and their intensity also changes. Let’s also keep in mind how precise a forecast needs to be, to be truly useful. After all: what good to you is a huge 4-hour-long solar storm that indeed hit Earth after 27 days, if it shifted by 6 hours and arrived to where you’re located in the middle of the day… You’re not going to see that aurora anyway…
3-day forecast
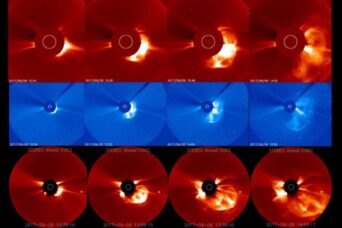
Photos of the Sun’s corona taken with the coronagraph. [source: NOAA]
Thanks to solar coronographs, we can observe the space above the Sun’s surface (the solar corona). For the coronal ejections of matter observed there, we can also estimate if and possibly when the particles ejected by them will reach Earth (or rather: when, if at all, Earth will fly into such a stream of matter).
In a similar way, we can also study “spots” on the Sun and predict the course of particle streams coming from this source. In NOAA’s 3-day forecast, Kp index values are shown in 3-hour intervals and to two decimal places. Unfortunately, both the strength and direction of the solar particles are so difficult to predict that this forecast too, despite its accuracy, is not overly reliable.
30-minute forecast
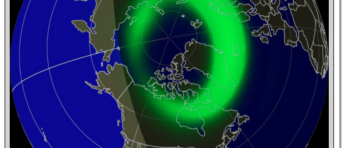
Northern Hemisphere aurora oval [NOAA]
Virtually the only reliable and accurate source of data on the strength and intensity of the solar wind flying toward Earth are satellites located at the so-called first Lagrange point (L1). This is, so to speak, the ‘windward’ counterpart of the ‘leeward’ L2 point, where the successor to the famous Hubble telescope – the infrared-operating JWST telescope – was recently placed.
This first Lagrange point is located about 1.5 million kilometers from Earth, directly facing the Sun, and thus in the path of the solar wind. Data from satellites there allows to predict magnetic storms in the Earth’s atmosphere quite accurately 15 to 45 minutes ahead. So it is an accurate forecast, but a very, very short one.
It is on the basis of this data that NOAA creates attractive animations of the aurora oval’s extent. But most of this animation is data for the past 24 hours. The actual, current forecast is just the last few frames.
Why in Iceland February is best for aurora viewing
Large magnetic storms occur in the Earth’s atmosphere throughout the year, but most often near the autumn and spring equinoxes – when the Earth’s axis is perpendicular to the Sun. So even though the night is the longest at the Winter Solstice (late December), the best time to see auroras is closer to the equinoxes, despite the fact that the nights are considerably shorter then.
But obviously the shorter the night, the less time we have to ‘catch’ the aurora and so the more lucky we need to be to actually succeed in it. In a sense the geomagnetic storm has a relatively small window of opportunity when it has to hit the atmosphere so we can see it. If it arrives a few hours early or late it will be daytime for us and we won’t notice its arrival nor the consecutive aurora.
So the best combination is somewhere in between when the night is the longest (late December) and when the magnetic storms are the strongest (late September and late March).
From this perspective it might be best to hunt for the aurora in the second half of October (about a month after the autumnal equinox) or the second half of February (about a month before the spring equinox). At those times of year the astronomical night in Iceland starts about 8 – 9 PM and lasts until 6 – 7 AM. So we have 10 to 12 hours of true dark night sky – still plenty and probably more than any of us would be willing to spend outside. In addition, the chance to see the morning aurora arrives in quite tolerable hours then 🙂
But that’s not all of it. Let’s look at the statistical data on Icelandic weather (graph below). We can see that although the trip in October (statistically) should be nicer and more mild, it is in February that we have a better chance of perfect conditions for admiring the northern lights.
In the chart below you can see data on the average level of cloud cover, wind strength, amount of precipitation and number of hours of sunshine for each month of the year (data for Reykjavik, averaged for the years 2000-2022).
As you can see, the average degree of cloud cover is fairly even throughout the year in Iceland. On a 9-point scale (from 0 to 8), it ranges only from 5.7 to 6.1, which is really quite high, but very stable. Rainfall totals are lower in spring and summer, and very high in September, but from October to March they are also quite stable. What may draw your attention is that although the average wind speed is lowest in the summer, it is clearly higher in the first quarter of the year than in the fourth. This is the effect of the famous Icelandic winter storms.
Sunlight hours, cloud cover, precipitation and wind in Reykjavik, 2000-2022.
Averages compiled from Veðurstofa Íslands raw data, vedur.isStormy weather tends to create extreme conditions – including extreme cloud cover. That’s why, if you move your cursor over the chart, you’ll see that the average daily sunshine hours in February were 2.3, in March 3.5, in October 3.1, and in November only 1.3. (As an aside: sunshine hours, of course, require that the sun not be obscured by clouds, which is why there are never many of them in Iceland, even though in June the day lasts almost 24 hours.)
Of course, when hunting for the aurora, we avoid sunny hours. However, since the number of sunny hours in February is more than 75% greater than in November, this means that it is much more common to see the sun in February, so we also have cloudless weather more often. Note that the length of the day is very similar in both months, as is the average level of cloud cover (5.8 vs. 5.7). Since the average cloud cover is the same in February, yet there are more hours of sunshine, this must mean that clouds are less frequent, but more intense when they do arrive.
In other words, if you come to Iceland in February there are high chances of great aurora-hunting weather. It might be cold and it will likely be windy, but you can count on a lot of days (and more importantly: nights) with no cloud cover. If you happen to arrive when a storm is coming, you’re out of luck and just have to wait it out. But after a few stormy days the skies should be clear again.
Conversely if you arrive in October/November time frame, the chances for a (strong) storm are lower, but you’re much more likely to find endless overcast days. Overall the weather might be more mild, but the skies will more often be hidden behind a thick cloud carpet with zero chances of seeing the aurora, even if the Kp index is at 9. So all in all, at least from the aurora hunting perspective, winter months are definitely better. Doesn’t mean you can’t see northern lights in Iceland in October through December. You can and a lot of people do. It’s just that your chances for that are somewhat lower then.
S
torms in Iceland can be very dangerous, but they happen not only in winter. The last catastrophic storm occurred on September 25 (sic!) 2022 in the East of the country and destroyed many homes and cars there (see
this article (in Icelandic only)). There is no strict rule that dangerous storms are a winter affair only.
Bibliography
To prepare the article, we used extremely extensive information from the following sources:
- https://www.gfz-potsdam.de/en/
- https://webb.nasa.gov
- https://www.gi.alaska.edu/
- https://www.swpc.noaa.gov/
- https://www.vedur.is/